Air transportation safety investigation report A21Q0097
Controlled flight into terrain
Synergy Aviation Ltd.
Guimbal Cabri G2 (helicopter), C-GSYN
Wachigabau Lake, Quebec
The Transportation Safety Board of Canada (TSB) investigated this occurrence for the purpose of advancing transportation safety. It is not the function of the Board to assign fault or determine civil or criminal liability. This report is not created for use in the context of legal, disciplinary or other proceedings. See Ownership and use of content.
-
Table of contents
Summary
On 08 October 2021, at 1030 Eastern Daylight Time (EDT), Synergy Aviation Ltd. Guimbal Cabri G2 helicopter (registration C-GSYN, serial number 1176) departed from a staging area in Chapais, Quebec, 12 nautical miles west of the Chibougamau/Chapais Airport (CYMT), Quebec. The pilot was the sole occupant on board the aircraft, which headed southwest toward Wachigabau Lake, Quebec, to perform airborne geophysical survey work.
At approximately 1144 EDT, as the helicopter was operating at low altitude over Wachigabau Lake, it struck the surface of the lake, overturned, and started to sink. The pilot sustained serious injuries from the impact, but conducted an underwater egress and swam to shore. No emergency locator transmitter distress beacon signal was captured by the Cospas-Sarsat system. At 1402 EDT, the Synergy Aviation Ltd. Operations manager called the Joint Rescue Coordination Centre in Trenton, Ontario, to report the accident. The pilot was rescued by a Canadian Armed Forces helicopter at 1641 EDT and taken to the Chibougamau Hospital.
1.0 Factual information
Transport Canada (TC) defines controlled flight into terrain (CFIT) as:
[a]n occurrence in which an aircraft, under the control of the crew, is flown into terrain, water or an obstacle with no prior awareness on the part of the crew of the impending disaster. Footnote 1
1.1 History of the flight
On the morning of 08 October 2021, the Synergy Aviation Ltd. (Synergy) Guimbal Cabri G2 helicopter was located at a staging area in Chapais, Quebec, 12 nautical miles (NM) west of the Chibougamau/Chapais Airport (CYMT), Quebec. The pilot would be performing aerial work for a client, Novatem Inc. (Novatem), a Canadian company that specializes in airborne geophysical surveys. Footnote 2
That morning, the pilot was tasked with conducting a low-level geophysical survey in the vicinity of Wachigabau Lake, Quebec, located 56 NM southwest of CYMT. The task included 2 survey flights with refuelling stops and a transit flight to Amos/Magny Airport (CYEY), Quebec, for helicopter maintenance. After a brief delay due to fog, the pilot informed his company’s flight follower of his intentions, donned his personal flotation device (PFD), and departed the staging area at 1030. Footnote 3 The pilot was alone on board the helicopter. The portion of the flight to the survey block, Footnote 4 which was flown between 500 and 1000 feet above ground level (AGL), was uneventful.
The pilot arrived at the survey block at around 1113. The area surrounding Wachigabau Lake is uninhabited and required the pilot to fly over both heavily forested areas and large bodies of water. The pilot descended to the operating height, which was below 100 feet AGL, positioned the helicopter on the western edge of the survey block, and began flying north-south track lines. To ensure the accuracy of the spacing between each line, the pilot relied on the Novatem aeromagnetic survey monitor. As the aircraft moved eastward within the survey block, the survey grid lines required flight over large areas of the lake. The helicopter’s recorded flight data points and estimated flight path are presented in Figure 1.
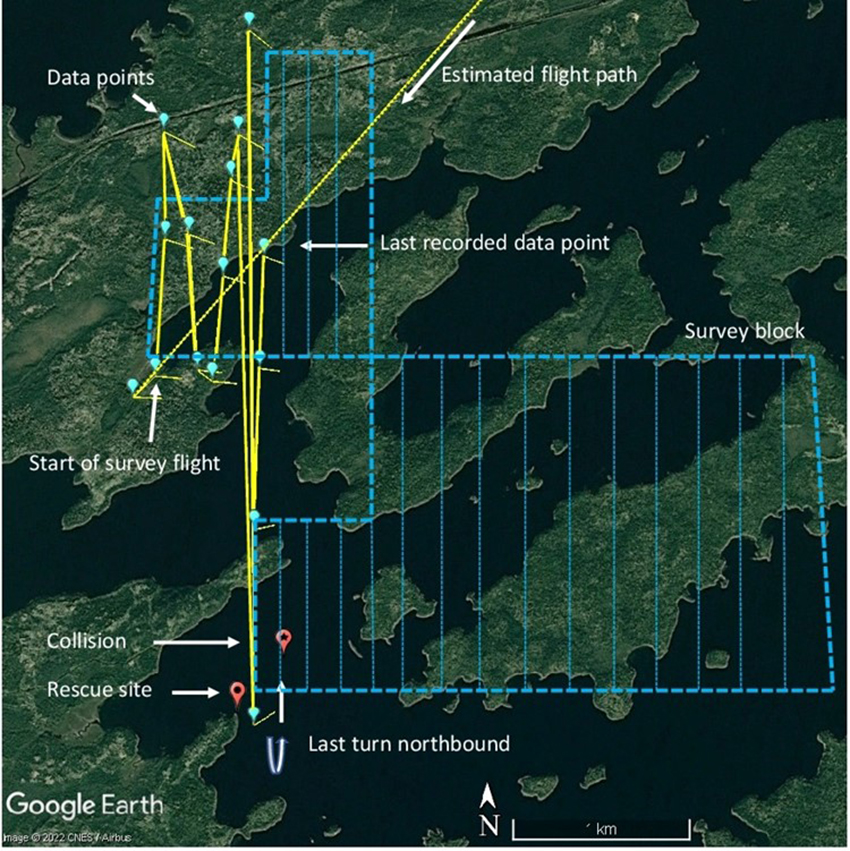
* Due to the tight spacing of the survey legs, it is difficult to depict the estimated flight path after the last recorded data point. However, the investigation did confirm that the helicopter kept flying to the southern edge of the block, conducted a turn, and then proceeded northbound.
At 1144, the pilot had completed a left turn over water approximately 1200 feet from the western shoreline and was beginning to fly a northbound leg. The pilot manoeuvred the helicopter to correct the track being flown to ensure proper alignment and lateral spacing on the leg. During this course correction, the helicopter descended, struck the surface of the lake, and overturned. The emergency flotation equipment did not activate, and the float containers did not inflate; the cockpit immediately flooded with water and the helicopter began to sink.Footnote 5 The pilot’s PFD automatically inflated underwater while he was still wearing his 4-point lap strap and shoulder harness. Despite the pilot’s initial shock and surprise, and even though his mobility was restricted with the inflated PFD, he was able to unbuckle and untangle himself from the lap belt and shoulder harness, disconnect the helmet communication cord, and egress the helicopter.
After reaching the water’s surface, the pilot grabbed his flight bag and helmet bag, which were floating close to him, and started swimming to the nearest shore, which was over 1400 feet away. After approximately 40 minutes, the pilot reached the shore and began survival activities, such as making a fire and drying his clothes and cellphone. The survival gear had sunk with the helicopter.
At 1243, the pilot’s cellphone had dried sufficiently to take photos. At 1322, the pilot received text messages from Synergy’s operations manager and its flight follower, as well as the technician from Novatem. The pilot responded and informed them of his situation.
At 1402, the Synergy operations manager notified the Joint Rescue Coordination Centre (JRCC) in Trenton, Ontario, which initiated a search and rescue (SAR) operation. The pilot was located at 1610 by a CC-130 Hercules aircraft. At 1641, a CH-146 Griffon helicopter rescued the pilot and took him to the Chibougamau Hospital.
No emergency locator transmitter (ELT) distress beacon signal was captured by the Cospas-Sarsat system.
1.2 Injuries to persons
The pilot was alone on board the helicopter. Table 1 outlines the degree of injuries received.
Degree of injury | Crew | Passengers | Persons not on board the aircraft | Total by injury |
---|---|---|---|---|
Fatal | 0 | – | – | 0 |
Serious | 1 | – | – | 1 |
Minor | 0 | – | – | 0 |
Total injured | 1 | – | – | 1 |
1.3 Damage to aircraft
The helicopter sank and was not recovered. Therefore, the extent of the damage to the helicopter is unknown.
1.4 Other damage
Fuel and oil may have been released into the lake; however, the exact quantity of each could not be determined.
1.5 Personnel information
Pilot licence | Commercial pilot licence - helicopter |
---|---|
Medical expiry date | 01 April 2022 |
Total flying hours | 407 |
Flight hours as pilot-in-command | 322 |
Flight hours on type | 235 |
Flight hours in the 7 days before the occurrence | 17.6 |
Hours on duty before the occurrence | 3.5 |
Hours off duty before the work period | 13 |
The pilot held a commercial pilot licence – helicopter that he had obtained through Synergy Flight Training Inc. in October 2018. He held a valid medical certificate and was endorsed to fly Bell 206, Guimbal Cabri G2, and Robinson R44 helicopter types. He worked for another helicopter company for 16 months before being hired as a junior pilotFootnote 6 at Synergy in July 2020.
His recurrent training on the Guimbal Cabri G2 was performed on 30 March 2021, and his pilot proficiency check had taken place on 29 September 2021 on a Robinson R44. The pilot held the appropriate licence and qualifications for the flight in accordance with existing regulations.
In April 2021, the pilot had deployed for the first time to support the same contract held between Synergy and Novatem. At that time, he had approximately 240 total flying hours and flew an additional 74 hours as part of the deployment. At the time of the occurrence, which was his second deployment for the contract, he had accumulated 407 hours total flying hours, including 322 hours as pilot-in-command.
Based on a review of the pilot’s work and rest schedule, there was no indication that the pilot’s performance was degraded by fatigue.
1.6 Aircraft information
1.6.1 General
Manufacturer | Hélicoptères Guimbal |
---|---|
Type, model and registration | Cabri G2, C-GSYN |
Year of manufacture | 2016 |
Serial number | 1176 |
Certificate of airworthiness/flight permit issue date | 01 December 2017 |
Total airframe time | 2227 hours |
Engine type (number of engines) | Lycoming O-360-J2A (1) |
Rotor type (number of blades) | Fully articulated, semi-rigid (3) |
Maximum allowable take-off weight | 1543 lb (700 kg) |
Recommended fuel type(s) | AVGAS 100LL – UL91, Premium MOGAS |
Fuel type used | AVGAS 100LL |
The occurrence helicopter was manufactured by Hélicoptères Guimbal in France in 2016. It was imported to Canada and the Cabri G2 received a TC type certificate in February 2017. The aircraft is a 2-seat, single-engine, piston-powered helicopter with an endurance of approximately 4.5 hours. Key features include a fully composite structure; a 3-bladed fully articulated, semi-rigid main rotor with composite blades; a shrouded tail rotor; a crash-resistant fuel cell; and energy-absorbing crash-resistant seats (Figure 2).
The aircraft’s journey log was not recovered. The technical records that were reviewed did not reveal any recorded deficiencies before the occurrence. The helicopter was maintained under a TC-approved maintenance schedule. The last copied pages of the journey log available at the company indicated that a 50-hour inspection was carried out on 22 September 2021. The records also indicated that the weight and centre of gravity were within prescribed limits. The occurrence pilot did not report any malfunctions or defects during the occurrence flight.
1.6.2 Novatem aeromagnetic survey equipment
The Novatem aeromagnetic survey equipmentFootnote 7 included an analyzer box, a battery, a keyboard, a monitor, and a magnetometer sensor that was placed in a boom (also called a stinger) and mounted on the nose of the helicopter. The installation of the stinger and its structural provisions was approved by Supplemental Type Certificate (STC) SH20-14, issued to Novatem by TC on 01 May 2020. Although the stinger had both an internal and an external global positioning system (GPS), and displayed GPS altitude in meters above sea level (ASL), it did not display height above ground because the helicopter was not equipped with a radar altimeter or a laser.Footnote 8
A conventional barometric altimeterFootnote 9 was the only instrument on board that provided a useable indication of the helicopter’s altitude.Footnote 10 The Novatem aeromagnetic survey monitor, mounted to the right of the console directly in front of the pilot, displayed the survey blocks and flight tracks (Figure 3).

The analyzer box, the battery, and the keyboard were placed in the space normally taken up by the left seat, which was removed for all aeromagnetic survey flights.
1.6.3 Emergency flotation equipment
The occurrence helicopter was also fitted with emergency flotation equipment manufactured by Hélicoptères Guimbal. The system’s components include float containers and an immersion detection electronic circuit designed to inflate the floats automatically (without any pilot input) when an immersion is detected, if the system is armed with the arming switch (Figure 4).
![Lower instrument panel showing the arming switch and light for the floats (Source: Guimbal, Service Bulletin SB 15-004 D: Pop-out Floats Capability 2 Installation [07 February 2016], p. 4/16, with TSB annotations)](./images/a21q0097-figure-04.jpg)
The installation of the emergency flotation equipment met the standards to operate a helicopter configured as a land aircraft over water.Footnote 11
1.7 Meteorological information
The graphic area forecast (GFA), issued at 0740 and valid as of 0800, forecast the following weather conditions for the Chibougamau area:
- clear sky and visibility greater than 6 statute miles (SM);
- patches of fog with visibility as low as ½ SM, ceilings at 200 feet AGL with tops at 1000 feet AGL; and
- locally, visibility as low as ⅛ SM in fog with ceilings down to the ground.
The GFA valid as of 1400 indicated clear skies and visibility greater than 6 SM. It did not forecast the fog that had previously been reported by the pilot.
The closest aviation weather reporting station was CYMT (56 NM northeast of the occurrence site). The aerodrome routine meteorological report issued at 1000 indicated the following:
- Winds from 110° true at 3 knots
- Visibility 9 SM
- Few clouds at 200 feet and broken ceiling at 3700 feet AGL
- Temperature 10 °C and dew point 10 °C
- Altimeter setting 30.20 inches of mercury
At the time of the occurrence, there was no fog in the vicinity of Wachigabau Lake, the winds were calm, and the visibility was unlimited. Due to the calm winds, glassy water conditions were present. The U.S. Federal Aviation Administration (FAA) provides the following caution with respect to calm wind conditions: “the smooth water surface presents a uniform appearance from above, somewhat like a mirror. This situation eliminates visual references for the pilot and can be extremely deceptive.”Footnote 12
A photo taken by the occurrence pilot approximately 1 hour after the accident shows clouds, good visibility, and a smooth reflecting water surface with patches of glassy water (Figure 5).
1.8 Aids to navigation
Not applicable.
1.9 Communications
Not applicable.
1.10 Aerodrome information
Not applicable.
1.11 Flight recorders
The helicopter was not equipped with a flight data recorder or a cockpit voice recorder, nor was either required by regulation.
The helicopter was, however, equipped with Spidertracks, a satellite flight-tracking system that records the following parameters every 2 minutes: GPS position, altitude ASL, date, time, ground speed, and direction of flight. Recorded data points were plotted to show an estimated flight path (as shown in Figure 1). The average ground speed recorded during the final 31 minutes of the flight was 59 knots. The altitudes recorded varied depending upon whether the helicopter flew over land or water. The average heights recorded during the final 31 minutes of the flight were 124 feet over land Footnote 13 and 54 feet over water. The lowest recorded heights were 77 feet over land and 26 feet over water.
A review of the recorded Spidertracks data from previous aeromagnetic survey flights conducted by other pilots showed a similar pattern of flying from 60 to 80 feet over land and from 20 to 40 feet over water.
1.12 Wreckage and impact information
After the accident, there were 2 attempts to locate the helicopter, but both were unsuccessful. On 04 May 2022, the aircraft insurer notified the TSB that no further attempts to locate or recover the helicopter were planned.
1.13 Medical and pathological information
According to information gathered during the investigation, there was no indication that the pilot’s performance was affected by medical factors.
1.14 Fire
Not applicable.
1.15 Survival aspects
1.15.1 Helmets, lap straps, and shoulder harnesses
The helicopter was equipped with lap straps and shoulder harnesses as required by section 702.44 of the Canadian Aviation Regulations (CARs). The pilot was wearing his lap strap and shoulder harness. He was also wearing a helmet, even though one was not required by regulation or by the operator.
1.15.2 Personal flotation devices
With respect to requirements and standards for the use of flotation devices in aviation, the CARs state:
No person shall conduct a take-off or a landing on water in an aircraft or operate an aircraft over water beyond a point where the aircraft could reach shore in the event of an engine failure, unless a life preserver, individual flotation device or personal flotation device is carried for each person on board.Footnote 14
PFDs on board aircraft must meet minimum performance standards and requirements. The applicable standards for PFDs for use in aviation are listed in CARs Chapter 551. The criteria for acceptance identify the requirement of a “manual only inflator” (Table 4).
Standard* | Criteria for acceptance for installation |
---|---|
ANSI/CAN/UL-12402-5:2015, Personal Flotation Devices – Part 5: Buoyancy Aids (Level 50) – Safety Requirements, First Edition, dated December 31, 2015, or later amendment. (effective 2021/05/24) | Acceptable, provided that the PFD has a performance level of Level 70, is not made of inherently buoyant material and is fitted with a manual only inflator [emphasis added]. |
UL 1180, Fully Inflatable Recreational Personal Flotation Devices, Second Edition, dated February 13, 2009, or later amendment. (effective 2021/05/24) | Acceptable, provided that the PFD meets the level of performance of a Type III and is fitted with a manual only inflator [emphasis added] and modified as per TP 14475, Edition 1, Chapter II, section 2.6.4. |
* Other acceptable standards include Canadian Technical Standard Order (CAN-TSO) C13f: Life Preservers, CAN-TSO C72c: Individual Flotation Devices, and Standards Council of Canada / Canadian General Standards Board CAN/CGSB-65.11-M88, CAN/CGSB-65.15-M88, CGSB-65-GP-15M, and CGSB-65-GP-11.
The PFD worn by the occurrence pilot was an inflatable Mustang model MD 3157 PFD with hydrostatic inflator technology that was designed to inflate automatically when submerged in 4 or more inches of water. Synergy had purchased PFDs that were marked TC-approved and they were selected based on information from the PFD manufacturer’s website. The PFD worn by the pilot inflated automatically after the impact, once water entered the cockpit and the helicopter began to sink.
The PFD’s identification tag (Figure 6) indicates that the PFD is “Transport Canada Approved” and lists the standard as CAN/CGSB- 65.7-2007, which is a marine standard.Footnote 15 The Mustang Survival data sheet indicates a TC Marine approval and refers to the same standard.Footnote 16 The owner’s manual indicates only that it is approved by TC, but refers to the Small Vessel Regulations.Footnote 17
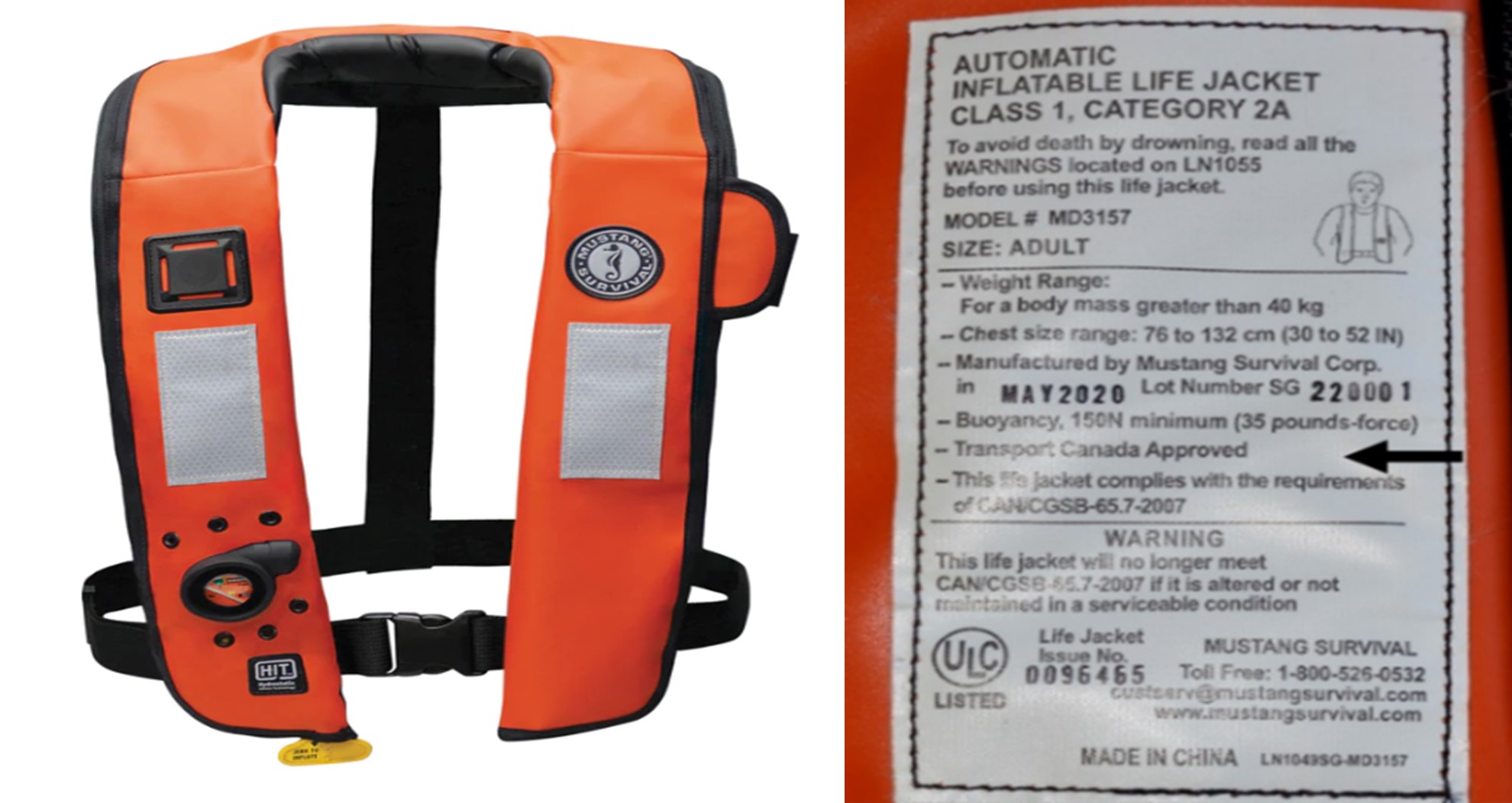
Once it was inflated, the PFD, which was positioned under the shoulder harness, exerted considerable pressure on the pilot’s chest, making the shoulder harness feel tighter; the inflated PFD also pushed up against the back of his helmet, pushing his chin down into his chest. Despite the pressure applied by the inflated PFD, the pilot was able to conduct a successful egress.
1.15.3 Underwater egress training
Underwater egress training provides pilots with the skills, knowledge, and confidence needed to survive real-life escape situations, such as a crash into the water or ditching.
Synergy coordinated underwater egress training for some pilots with a company specializing in safety and survival training. The occurrence pilot had received this training on 30 August 2021.
1.15.4 Flight following
1.15.4.1 Synergy operational control, flight following, and flight watch
Before the occurrence, Synergy had implemented a flight-following system, which included a GPS-based flight-tracking system, dedicated flight followers, and company procedures, even though only a Type D operational control systemFootnote 18 was required by regulation for their CARs Subpart 702 operations.
The duties of the flight followers included, among other things, monitoring aircraft with open operational flight plans every 30 minutes for “Ops Normal” (normal operations) status.Footnote 19
The procedures also contained directives for overdue and missing aircraft as well as what to do in the event of an aircraft accident. An aircraft is considered overdue if an “[a]ircraft signal has stopped moving and no Landed Safely, Landed Done For Day or Landed Safely Maintenance Required message has been received.”Footnote 20
Flight-follower duties were assigned to junior pilots hired by the company. On the day of the occurrence, a junior pilot was assigned flight-following duties, but was also scheduled to conduct a training flight. As a result, the flight follower was multi-tasking between conducting flight-following duties and preparing for the flight. The investigation could not determine whether the flight follower was monitoring the occurrence helicopter every 30 minutes while also completing other tasks.
1.15.4.2 Spidertracks
Spidertracks is a private company that provides equipment to support real-time flight tracking, automated flight watch, 2-way communication, and flight-data monitoring. The system offers various flight-tracking and communication methods. For example, a pilot can send an SOS message while flying by using a 3-button keypad, which is part of the system and installed inside of the cockpit.
The Spidertracks system’s instruction manual states:
The Spidertracks system comes with a two tiered emergency management framework, which are [sic] aligned with the ICAO [International Civil Aviation Organization] definitions of uncertainty and alert. Recipients of these alert notifications are fully customisable within the Organisational Settings of the website.Footnote 21
The manual also describes the system’s tracking modes:
Spidertracks provides both passive and active tracking capabilities (referred to as normal and watch modes respectively). Under normal tracking conditions, the Spider will report positional information and flight events in real time, however, if the aircraft were to encounter an emergency situation in flight, ground personnel would not be alerted to this unless there was a conscious SOS button pressed by the crew. In watch mode, the Spidertracks system is actively monitoring the status of the flight. If communication with the aircraft is lost for a period of ten minutes, a tier one alert will be pushed through to the emergency management framework.Footnote 22
After the impact, the occurrence helicopter’s positional information remained displayed, but no tier one alert was raised to the emergency management framework. A post-occurrence check of Synergy’s Spidertracks organizational settings found that the system had been in normal mode.
1.15.4.3 Accident notification and response
The Novatem project manager (located in Quebec), who had been provided access to Spidertracks, was watching the progression of the occurrence helicopter’s flight. Spidertracks displayed the helicopter’s last reported position at 1141.
At 1200, about 15 minutes after the accident, the project manager observed that the helicopter’s position had not changed. At 1246, he called Synergy (located in Alberta) to request an update on the helicopter’s flight status. After discussion and an examination of the Spidertracks system, it was determined that the system had ceased reporting the helicopter’s position. At approximately 1250, Synergy initiated its overdue aircraft procedures and sent text messages to the pilot at 1255 and 1258.
Synergy attempted, without success, to coordinate the SAR with other helicopter operators and called the JRCC in Trenton, Ontario, at 1402 to report the accident and request assistance.
1.15.5 Emergency locator transmitters
The helicopter was equipped with a Kannad 406 AF-Compact ELT (part number S1840501-01). No signal from the 406 MHz ELT was received by the Cospas-Sarsat system.
When an aircraft crashes into water, it is possible that the ELT will end up below the surface of the water before the 406 MHz signal is transmitted. In this occurrence, it could not be determined whether the absence of a signal was due to submersion in water, damage during the impact sequence, or because the ELT was not in the armed position.
1.16 Tests and research
Not applicable.
1.17 Organizational and management information
1.17.1 Novatem
1.17.1.1 General
Novatem is a Canadian company with experience in airborne geophysical surveying that conducts high-resolution magnetics, radiometrics, and electromagnetic surveys.Footnote 23 As a result of advances in electronics, Novatem miniaturized its equipment, and this new technology allowed the equipment to be installed into the occurrence helicopter’s stinger.
Given that the Guimbal Cabri G2 has less magnetic interference, lower operating costs, and lower fuel consumption than other helicopters, Novatem approached Synergy—the only Canadian commercial helicopter operator using the Guimbal Cabri G2 at that time—to conduct airborne geophysical surveys. Novatem and Synergy entered into an open-ended agreement, the Synergy / Novatem G2 Operating Contract, and fitted the Guimbal Cabri G2 with this new aeromagnetic equipment.
1.17.1.2 Novatem aeromagnetic survey equipment requirements
In order to obtain good-quality data, the survey has to be flown within certain limits that are inherent to the equipment that accompanies this new technology. The speed at which the survey is flown has no bearing on the quality of data obtained. However, the aircraft’s height does. To obtain quality data, the helicopter must consistently maintain the optimal height (approximately 25 m [82 feet] over land and water) on all survey lines.
This means that the helicopter is required to fly the contour of the terrain as much as possible (Figure 7). Novatem has stated that some variations in height due to obstacles and other safety concerns are acceptable, and data collected can be corrected with software calculations and extrapolation algorithms. While some pilots, including the occurrence pilot, had been briefed verbally by the chief pilot on the requirement to fly the contour of the terrain, the requirement to fly at and maintain the optimal height of 25 m (82 feet) was not clearly communicated. Neither the requirement to fly and maintain the optimal height of 25 m (82 feet) nor the requirement to fly the contour of the terrain was included in the Guimbal Aeromagnetic Survey Procedure (GASP) (see Section 1.17.2.3 Development of the Guimbal Aeromagnetic Survey Procedure of this report).
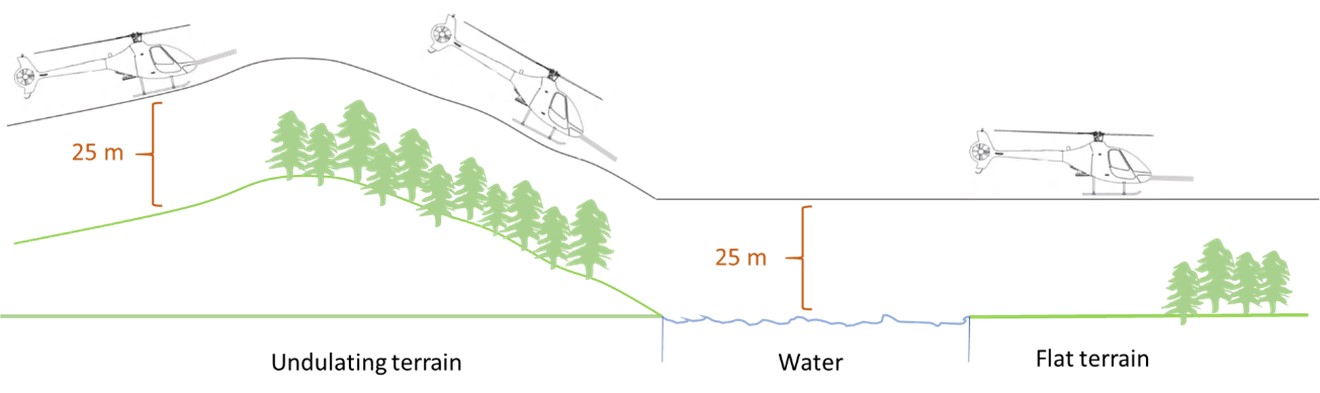
The aeromagnetic survey monitor provides some navigational data to assist the pilot when flying the survey block, such as survey line spacing (25 m [82 feet] apart), the lateral distance off the line, and the remaining distance to the end of the current survey line being flown (Figure 8).
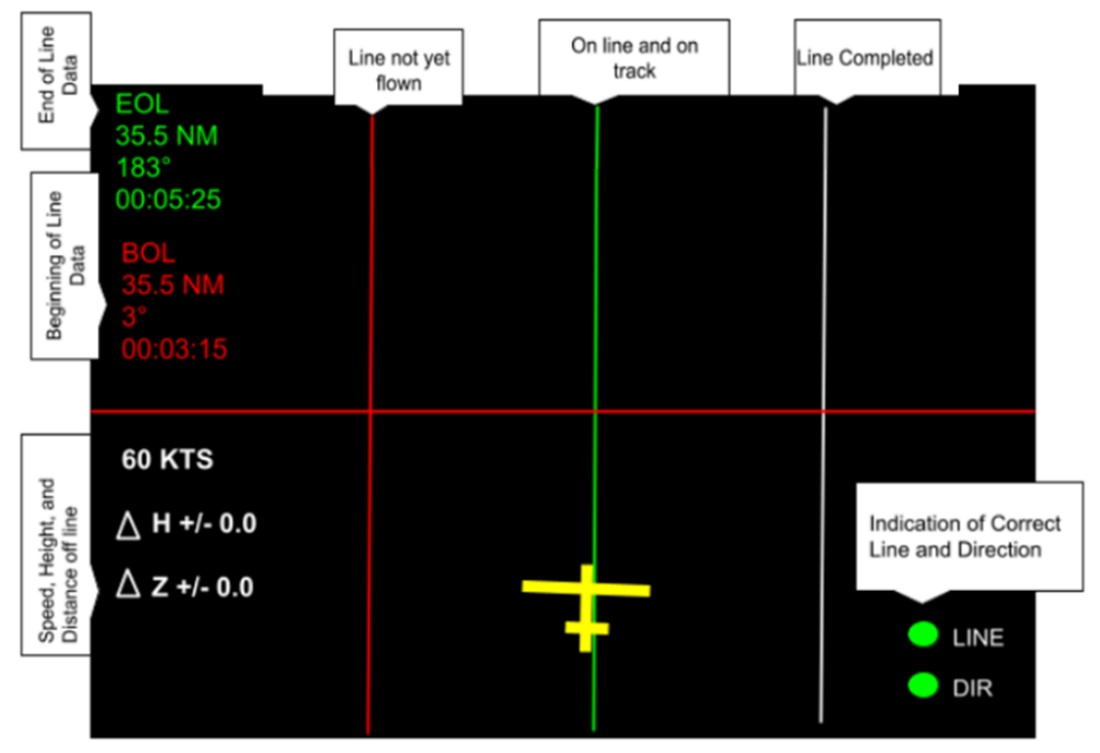
The information displayed on Novatem’s aeromagnetic survey monitor is meant to be a navigational aid to increase the precision of the flight profile, but it does not help pilots manage the risk of being too low if an inadvertent descent occurs. Thus, Synergy pilots did not have precise information to determine their height above the ground. In addition, there are no TC regulations or requirements for CARs Subpart 702 day visual flight rules (VFR) helicopter operations to require equipment that provides a means of alerting the pilot to the precise height of the aircraft above land or water, or to alert the pilot if the aircraft descends below a specific height.
During this contract, the recorded data was downloaded by Novatem once a flight was completed. Novatem then provided the pilots with feedback about the flight profile, particularly if the height flown needed to be adjusted.
Having completed 2 days of survey flying (on 05 and 06 October 2021), the occurrence pilot was informed by Novatem that the survey data for both days showed that he was, on average, 10 m (32 feet) above the survey heights flown by the previous pilot, and that the survey height should be lowered. After discussing the situation with the chief pilot, the occurrence pilot adjusted his flight profile by flying lower on 07 October. Novatem was then satisfied with the survey results from that day.
A review of the flight data from the Novatem aeromagnetic equipment indicated that pilots generally flew between 23 to 25 m (75 to 82 feet) over land with vegetation (trees) and 12 m (approximately 40 feet) over water or flat, open fields.
1.17.2 Synergy Aviation Ltd.
1.17.2.1 General
Synergy Aviation Ltd. has been in operation since 2014 at Edmonton/Villeneuve Airport (CZVL), Alberta. The company holds an air operator certificate under CARs subparts 702 (Aerial Work) and 703 (Air Taxi Operations) with a mixed fleet of fixed- and rotary-wing aircraft. The company’s focus is on aerial surveillance and pipeline integrity monitoring within the oil and gas sector. In order to take a proactive approach to a perceived pilot shortage in the industry, the company launched a flight training school in 2016.
1.17.2.2 Mentorship program and pilot experience levels
After completing the training program and obtaining a commercial helicopter pilot licence, some pilots from Synergy’s flight school are hired by Synergy as line pilots. There is a limited number of flying jobs for new pilots while they are building up their flying hours and experience. These new pilots are typically assigned charter, tourism, or ferry flights, because these types of flights typically do not have minimum flying hours and experience requirements.
In addition to the flight school, Synergy developed a mentorship program in which a junior pilot flies with a senior pilot during pipeline surveillance flights.Footnote 24 Under this program, and under the supervision of a senior pilot, junior pilots are exposed to operational flying and gain experience in flight planning, flying, operating procedures, and pilot decision making (PDM).
Pilot experience levels, measured in terms of total flying hours, are often seen as a mitigating safety measure for operators and clients when conducting operations under CARs subparts 702 and 703. Operators often require pilots to have a minimum number of hours or amount of experience; however, this requirement is sometimes set by the client. In some cases, operators indicate that they rarely hire pilots with a low number of flying hours.Footnote 25 The requirement for minimum total flying hours or operational flying experience seems to provide operators and clients with the confidence that the pilots chosen to conduct the specific work have the operational flying experience needed for the task.
Pilots with more experience are generally seen to display more operational and technical knowledge and more developed decision-making skills. They have more overall experience in flying in specific operating environments and in identifying hazards and risks. They are also better equipped to mitigate the hazards and risks in abnormal and emergency situations. Pilots with less experience, however, can be seen to display ineffective PDM when identifying or assessing risks or insufficient advanced knowledge of new operating environments, e.g., operating in a low-level environment, over water, or at night.
However, data from the TSB’s safety issue investigation (SII) into Canada’s air-taxi industryFootnote 26 indicates that, while a large proportion of inexperienced personnel in an organization is seen as posing a higher risk to safety, there was only one accident category (fuel-related accidents) in which pilots with relatively fewer flight hours were involved. In all other accident categories, the pilots involved had a higher average number of flight hours. When looking at experience in PDM, pilots on both ends of the experience spectrum were at risk for ineffective decision making, although for different reasons. Operators described inexperience as a factor that made it more difficult to identify and assess risks. However, as pilots gain more experience, their perception of risk will often change, making them more willing to take risks.
As part of this investigation, some randomly selected air operators with experience in conducting aeromagnetic surveys were interviewed. The operators indicated that they would typically only select pilots with a high number of flight hours or previous experience in conducting aeromagnetic surveys.Footnote 27
The Synergy / Novatem G2 Operating Contract did not stipulate a minimum number of pilot hours or level of experience. As a result, Synergy viewed this as an opportunity for its low-time pilots to gain operational experience and increase their total flying hours. The total flying hours for 5 of the 6 pilots supporting Novatem’s geophysical survey operation were reported as follows: 240, 242, 445, 830, and 2750 (these numbers were estimated at the start of their initial deployment).
1.17.2.3 Development of the Guimbal Aeromagnetic Survey Procedure
Synergy was contracted by Novatem to perform geophysical survey flights, even though Synergy had never performed this type of aerial work. Given their company’s operational background with the oil and gas industry, Synergy personnel assessed the geophysical survey flights in the same way as the surveillance flights they had previously conducted.
The Synergy chief pilot at the time designated one of the surveillance pilots to work with Novatem and learn how to perform aeromagnetic survey flights between 11 June and 28 July 2020 in the region of Abitibi-Témiscamingue, Quebec. In consultation with Novatem, this designated pilot developed the GASP.
While the GASP was being developed, Synergy assigned another pilot to be trained on site in Saskatchewan by the designated pilot and to fly trial flights over land from 05 August to 16 August 2020. This trial was used to validate the GASP and to provide Synergy with the confidence that the GASP was an effective tool that would provide pilots with the knowledge required to safely conduct the aeromagnetic survey task.
The GASP provided a general overview of the airborne geophysical survey operation as well as information on the Novatem aeromagnetic on-board equipment that was to be used, and on flight planning and hazard assessment.
Under the heading Safety, the GASP stated that “[h]azards to consider are wires, persons or livestock, trees, rising terrain, and water.”Footnote 28 However the document did not offer mitigations on how to deal with these hazards. Rather, it referred readers to the Exercise 22 – Low Level Operations of the Helicopter Flight Training Manual on TC’s website.Footnote 29
The GASP’s section on flight planning and hazard assessment listed 9 hazards to consider, including lakes, rising terrain, and tall standalone obstacles (e.g., trees), Footnote 30 but it did not provide clear guidance on how to assess and mitigate those hazards within the context of aerial work, aeromagnetic survey work, low-level flying, or over-water operations.
The GASP provided no information on contour flying and consistently maintaining the optimal height (25 m [82 feet]) flown on all survey lines—the most important parameter for obtaining quality data. In addition, there was no information or guidance on the precise vertical boundaries or recommended heights to be flown to help pilots meet the Novatem aeromagnetic equipment requirements to obtain valid data. The investigation revealed that the pilots needed to rely on post-flight feedback from Novatem staff to know if the height flown was low enough to provide valid data. During the flights, pilots had to rely on their own visual cues to judge and maintain height over terrain because the helicopter was only equipped with a barometric altimeter.
While the GASP did not include any limitation on heights or altitudes, following this occurrence, the GASP was amended to include the following statement: “All flights shall be conducted no lower than 200ft AGL.”[emphasis in original]Footnote 31
1.17.2.4 Pilot training
Developing and implementing training that is representative of the operational environment is a complex and challenging task for new or specialized operations. Pilots should be introduced to and educated on the aircraft, operating systems, and operational procedures, as well as associated hazards and risks of the operating environment, in a methodical and safe manner. While a training environment must offer pilots realistic training to prepare them for the operational environment in which they will fly and operate, exposing pilots to every kind of environment that they will face is not always possible.
A review of the company operations manual (COM) and the training syllabus revealed that they did not include dedicated training for the geophysical survey operation or a have specific section on low-level flying or over-water operations. However, the GASP states “[b]efore flying any type of survey work, you should have at least completed a low level flying course and low level emergency training.”Footnote 32 The low-level-flying course and low-level emergency training were part of the surveillance flight training; however, it did not include flying over water. The hazards created by glassy water conditions and strategies for mitigating the risk of CFIT were not discussed in either the COM or the training syllabus documents.
The hazards of glassy water conditions are not included in TC’s Helicopter Flight Training Manual, which is used for both private and commercial helicopter pilot licence training. In addition, there is very little guidance material from TC or the FAA regarding the hazards of glassy water conditions as they relate to helicopter operations. In Canada, TC provides some information concerning glassy water, but it is limited to the instructor guide for seaplane ratings.Footnote 33 In the United States, the FAA provides somewhat more detailed information, but again, it focusses on seaplane operations.Footnote 34
The occurrence pilot had some knowledge about glassy water conditions from informal discussions held during his initial flight training and throughout his pilot career; however, he had not been exposed to this hazard in actual flight operations or during geophysical survey (i.e., low level or over water) operations, and had no experience managing the hazard.
Having equipment like the Novatem aeromagnetic survey equipment or emergency floats installed on the helicopter during in-flight training help make the training more realistic and effective. However, the Novatem aeromagnetic survey equipment could not be installed in the helicopter for the training flights because it required that the left seat (where the instructor would sit) be removed. In addition, because the installation of the helicopter’s emergency flotation equipment required the aircraft to be out of service for some time, the over-water in-flight training was conducted before, and without the emergency flotation equipment installed.
The in-flight training for geophysical surveys was given in the vicinity of CZVL, which is surrounded by mainly open, flat land with few lakes. In contrast, the Chapais area, where the occurrence flight took place, has undulating, heavily forested terrain with several large lakes.
In an attempt to simulate the geophysical flight profiles, the in-flight training was performed at 200 feet above a runway, using the runway’s left edge, centreline, and right edge to simulate track spacing. This training did not include flights at heights that would be flown operationally (i.e., 25 m [82 feet]). As for the over-water training given to the occurrence pilot, it included a ground school discussion, mainly focusing on the use of PFDs. The in-flight training included a flight over a lake, but at heights of up to 2000 feet AGL because the emergency flotation equipment was not installed at the time. Because of the requirement for emergency flotation equipment to fly low over water, no low-level over-water in-flight training (below 200 feet or at the operational height of 25 m [82 feet]) was conducted.
1.17.2.5 Synergy Aviation Ltd.’s safety management system
Many operators, such as Synergy, are taking a proactive approach to safety, identifying and mitigating risks associated with their activities, and a number of these operators are taking measures that exceed regulatory requirements. No safety management system (SMS) regulations exist for CARs Subpart 702 operators; however, Synergy voluntarily introduced an SMS in 2017. Because the SMS was introduced voluntarily, it has never been the subject of a TC regulatory oversight or surveillance activity, nor was it required to be.
Synergy’s SMS manual indicates that data for its safety oversight component comes from employees submitting hazard and occurrence reports through an online reporting tool, by email, or from verbal reports to a supervisor, a manager, or the safety officer. New activities, such as using new equipment or new locations of operations, require a risk assessment and associated mitigation, monitoring, and follow-up, as indicated by the risk matrix and risk level assessed. The manual also indicates that occurrences, such as accidents, will result in a reactive occurrence report that will lead to an investigation, analysis, and risk management. Footnote 35
The investigation determined that before the occurrence, several pilots had verbally raised concerns with the chief pilot about the low heights required for survey flights. They deemed the flight profile risky and were often uncomfortable with these low heights.
The investigation did not reveal any entries or follow-ups in the online reporting tool from pilots concerning low-level flight or unsafe conditions in the geophysical survey operation. These concerns were managed informally with guidance to “fly as low as safe.” No risk assessments were found for the equipment used or for the new location for the Novatem aeromagnetic survey operation. However, after the occurrence, an SMS investigation and report were completed, and a series of corrective actions were identified. The company continues to monitor their implementation.
1.17.3 Transport Canada
1.17.3.1 Intentional low flying
The Transport Canada Aeronautical Information Manual (TC AIM) contains the following:
Warning—Intentional low flying is hazardous. Transport Canada advises pilots that low flying, especially for weather avoidance, is a high-risk activity. Footnote 36
The TSB has investigated several occurrences involving intentional low flying. Footnote 37
Intentional low flying is common within the aerial-work sector and increases the risk of an accident. It reduces the available height and window of time that a pilot needs to recognize and manage unexpected events or emergencies, such as engine failure, loss of control, or inadvertent descent.
Flight profiles in geophysical survey operations are inherently risky because aircraft are operated at low heights for extended periods of time. This requires constant attention to keep a steady heading and consistent height, typically achieved with the use of outside visual cues. Visibility, in terms of the distance a pilot can see ahead, can also be limited, which may further reduce visual cues. Furthermore, this particular operation can, at times, require pilots to focus their attention on the aeromagnetic survey monitor installed in the cockpit to ensure that the survey plot is flown accurately. Focusing attention inside the cockpit can detract from the external vigilance required for safety of flight.
Most aerial-work operations are conducted in day VFR conditions using light aircraft, where the standard barometric altimeter is the only equipment required by regulation that provides altitude information. Footnote 38 However, standard barometric altimeters are typically not set to provide information on height above the ground; they normally provide altitude with respect to mean sea level. In addition, a barometric altimeter has no means of alerting a pilot when the aircraft descends below the prescribed height or altitude. To address this issue, the use of radio altimeters for geophysical survey operations is recommended by industry best practice. Footnote 39
1.17.3.2 Company operations manual
Section 702.81 of the CARs Footnote 40 states that every air operator that operates under CARs Subpart 702 shall establish and maintain a COM that meets the requirements of section 702.82 of the CARs, that the COM shall be submitted to TC, and that if there are any changes to the company’s operations, the operator shall amend its COM. The COM’s content requirements are listed in the Commercial Air Service Standards (CASS). If the CASS requirements are met, TC shall approve the COM and any amendments to it. In addition, subsection 702.82(1) of the CARs states the following:
A company operations manual, which may be issued in separate parts corresponding to specific aspects of an operation, shall include the instructions and information necessary to enable the personnel concerned to perform their duties safely and shall contain the information required by the Commercial Air Service Standards. Footnote 41
CASS 722.82(2) lists the items a CARs Subpart 702 operator that conducts day VFR operations is required to include in its COM. In particular, paragraph 722.82(2)(y) requires:
[P]rocedures related to aerial work operations including, as applicable:
- carriage of external loads;
- low level flight precautions;
- towing precautions, pick-up and release procedures;
- helicopter external load procedures, including flight and ground crew signals and briefing procedures, steps to be taken before starting an external load operation, hazards of oscillating loads, low density loads and unfamiliar load configurations; and
- operational restrictions related to aerial work operations.
Footnote 42
The GASP was not incorporated into the Synergy COM and, as a result, TC was unable to provide oversight. A review of the COM revealed that there was no additional information on procedures, precautions, or operational restrictions related to geophysical survey operations. There was minimal information concerning low-level flight. Footnote 43 Information concerning over-water flight precautions was limited to Section 4.24 Operating Over Water (Land Aircraft) and required that aircraft be equipped with approved emergency flotation equipment, that pilots maintain direct flight following, and that a flight plan or flight itinerary and company operational flight plan be filed. However, the COM also stated the following:
Pilots shall operate the helicopter at an altitude that will provide adequate time during autorotation descent for full inflation of the floatation devices prior to water contact. Footnote 44
1.17.3.3 Safety management systems
SMS regulations have yet to be implemented in the following areas: commuter services, air taxi, aerial work, other approved maintenance operations, aircraft manufacturing, aircraft certification, and flight training. These segments together comprise approximately 800 air operators representing more than 90% of Canadian certificate holders. Although not required by regulation, many companies within these groups have voluntarily chosen to implement SMSs. However, TC does not monitor or regulate SMSs for these operators.
Following the TSB investigation into a fatal helicopter accident in 2013, Footnote 45 the Board recommended that
the Department of Transport require all commercial aviation operators in Canada to implement a formal safety management system.
TSB Recommendation A16-12
Since the publication of this recommendation, there have been several responses from TC. In its October 2022 response, TC stated that it agrees with the recommendation. TC has continued to advance Phase II of its SMS policy review, which will examine expansion of SMS to other aviation sectors, and work will continue until such regulations come into effect. To this end, TC presented an update on its policy review, options for a proposed regulatory approach, and timelines for modernization at the 19th Canadian Aviation Regulation Advisory Council (CARAC) plenary, which took place from 23 to 25 November 2021.
Given the scale of this initiative, TC has divided its modernization of the SMS framework into 2 packages. TC is currently drafting Package One, Notice of Proposed Amendment (NPA) for SMS Modernization. Package One proposes to extend SMS to design and manufacturing organizations. Also, it will propose compliance and harmonization of Canadian regulations with international standards. Following TC’s response in October 2022, it has confirmed that the NPA will be published on the CARAC website in Spring 2023. Publication in the Canada Gazette, Part I is planned for mid-2024.
Package Two will propose to examine the expansion of SMS to other sectors not currently covered under the SMS CARs requirements. Timelines for Package Two remain in development.
The issue of safety management has been on the TSB Watchlist since 2010. As highlighted in Watchlist 2022, progress has been slow to extend the application of SMS beyond CARs Subpart 705 operators. While the Board is encouraged that TC is conducting an SMS policy review that will assist in developing recommendations to modernize and expand SMS requirements, until this review is complete and changes are implemented to the SMS requirements, it is unclear whether these efforts will address the safety deficiency identified in the recommendation. In March 2023, the Board considered TC’s response to Recommendation A16-12 to be Satisfactory in Part. Footnote 46
1.18 Additional information
1.18.1 Glassy water conditions
Glassy water can be described as follows:
[a]s a fluid, water seeks its own level, and forms a flat, glassy surface if undisturbed. […] Under calm wind conditions, the smooth water surface presents a uniform appearance from above, somewhat like a mirror. This situation eliminates visual references for the pilot and can be extremely deceptive.” Footnote 47
The visual aspects of glassy water make accurate depth perception very difficult. The “flat, featureless surface makes it far more difficult to gauge altitude accurately, and reflections can create confusing optical illusions.” Footnote 48 The presence of calm surface winds over water can result in glassy water conditions. Glassy water conditions make it difficult for pilots—even experienced pilots—to judge the aircraft’s height above the water given the absence of surface features and visual references. As well, the smooth, reflecting surface can lead to confusing illusions as clouds or shore features are reproduced in stunning detail and full colour. Footnote 49
On the morning of the occurrence flight, glassy water conditions were present due to the light surface winds in the area.
1.18.2 Human performance
1.18.2.1 Visual cues and depth perception
Visual cues are essential for depth perception. The quality and number of visual cues in the environment are important because they allow pilots to judge height, distance, spacing, and closing speeds to and from obstacles.Footnote 50 During a flight over water, waves, crests, and foam provide light and shadow, and texture gradients. While trees and a shoreline can also provide visual cues, the combination of distance and height while moving over water can interfere with correct depth perception.Footnote 51
When a pilot flies over glassy water, the reduction of visual cues in the immediate field of view may contribute to a loss of depth perception. This can go undetected because even when visibility is good, the cues for perceiving depth could be diminished. Glassy water conditions present a significant risk of collision with the water because they can lead to inadvertent altitude deviations, such as undetected climbs or descents.
1.18.2.2 Single-pilot crew resource management in helicopter operations
Crew resource management (CRM) is the effective use of all resources, including crew members, aircraft systems, supporting facilities and persons, to achieve safe and efficient operations.Footnote 52 The objective of CRM is to enhance communication, interaction, human factors, and management skills of the crew members concerned. In Advisory Circular 700-042: Crew Resource Management, TC recommends that aerial-work and air-taxi operators conduct single-pilot resource management (SRM) training. More specifically, the Advisory Circular states:
SRM adapts concepts from CRM to the single-pilot environment. […] Current CRM training concepts include threat and error management (TEM), which speaks to the identification and analysis of potential hazards; the implementation of appropriate strategies to handle threats; and the implementation of steps to avoid, trap, or mitigate errors before they lead to undesired consequences such as an undesired aircraft state.Footnote 53
The Advisory Circular also states that SRM training should be focused on areas relevant to the single-pilot and to the operation. It recommends that, among other topics, training should include situational awareness, workload management, decision making, and surprise and startle effect.Footnote 54
The occurrence pilot took a PDM course and received CRM training on 09 January 2019. The investigation did not determine whether the CRM training included SRM training as defined by TC.
1.18.3 Operational pressures
Air operators face a number of operational pressures—pressures that can originate from various sources such as service providers, clients, customers, and the flying environment. These pressures must be identified and managed for air operators to deliver a service, stay safe, and remain economically viable. The investigation determined that the following operational pressures were present in this occurrence:
- Novatem’s requirement to obtain quality data: Novatem’s instruction for pilots to fly lower, or as low as possible, was motivated by the need to record good-quality data. Without the integration of a precise height indication on board, the pilots were left to visually estimate and rely solely on feedback from Novatem to determine whether they had flown a proper flight profile at the correct height. This also left them to determine and maintain their height using visual cues only.
- Synergy’s direction to fly low: The communications between the company and its pilots to fly as low as safely possible while flying the contour of the terrain within their own personal comfort limits was motivated by the need to ensure Synergy met Novatem’s expectations.
- Relative low number of flight hours/inexperience of the pilots: Novatem did not require a minimum amount of total flight time or pilot experience because it believed Synergy would provide qualified and experienced pilots. Without a limitation in place with respect to the minimum amount of total flight time or pilot experience required, Synergy could select junior pilots, providing them the opportunity to gain experience. In addition, these pilots were deployed to another province and, under the Type D operational control system, were basically left to operate alone and deal directly with the client. Operating alone can leave pilots with few resources to assist in decision making, identifying hazards and risks, or trying to select mitigating options or courses of action, especially when those pilots have low levels of experience.
- Pilots’ individual pressure: The pilots were motivated to complete the tasks and to meet the client’s and the employer’s expectations. This pressure can often push pilots to operate beyond their own comfort levels.
1.18.3.1 Acceptance of unsafe practices
Operational pressures may lead operators and their pilots to accept unsafe practices. The acceptance of unsafe practices can be characterized as changes—whether adopted, unintentional, or unnoticed—in operations that lead to a migration from safe operations to operations that involve risk. These changes can often pass as undetected drift from safe practices and may gradually become accepted as part of the job. When unsafe practices continue with positive outcomes (such as successful flights or satisfied customers), or with no negative outcomes (such as no incidents or accidents), accepting these practices as safe can seem rational, logical, and easy to justify. Eventually, they become accepted as the norm by the operator’s management and seasoned pilots, and are even taught to new hires.
In this occurrence, an example of an accepted unsafe practice was not flying a consistent contour profile over terrain, which resulted in flying below the operational height required for the survey flights.
1.18.4 Safety measures to mitigate operational pressures
Safety measures are mitigations that can counter operational pressures. These include company policies and procedures, aircraft modifications, training, and safety equipment. Synergy implemented several safety measures to deal with this new operation:
- Policies and procedures: created the GASP and implemented a flight-following system.
- Helicopter modifications: installed emergency flotation equipment for over-water operations.
- Training: provided ground and in-flight training on topics such as underwater egress, flying survey profiles, helicopter modifications, and safety equipment.
- Safety equipment: provided safety equipment, such as PFDs.
Synergy also addressed operational pressures in its ground training by educating their pilots on the information contained in its company policy manuals. A training presentation on avoiding inadvertent flight into instrument meteorological conditions listed the following types of operating pressures that can affect PDM:
Company: Pressure to complete tasks
Customer: Pressure to please the customer in spite of safety
Self: Desire to accomplish more than is reasonable
Peers: Pressure to perform at the perceived level of others (culture)
External: Distractions from the job at hand (family/finances/personal)Footnote 55
Client pressure was also addressed in the Helicopter Flight Policies manual:
The PIC [pilot-in-command] is responsible for the safe operation of all flights. The PIC has the final say for all phases of flight operations. Should there be any pressure or dispute from a client without resolving the dispute, the PIC shall terminate the flight and contact management in order to resolve the dispute. At no point shall a pilot feel pressured during any phase of flight operations to do anything they are not comfortable with.Footnote 56
1.18.5 Mitigating strategies and industry initiatives in aerial work
Organizations such as the International Airborne Geophysics Safety Association (IAGSA) and the Flight Safety Foundation (FSF) offer recommendations and safe operating practices to members and operators conducting aviation operations. These recommendations span a range of issues such as company directives, standard operating procedures, pilot training, and aircraft selection and configuration, as well as equipment modifications.
1.18.5.1 International Airborne Geophysics Safety Association
IAGSA promotes safety in airborne geophysical operations by, among other things, developing recommended practices, providing a risk assessment template for operators when they start an operation, serving as a centre for the exchange of safety information, and providing a repository for specialized statistics. The recommended practices for airborne geophysical survey operations are available online and target operating standards, training, experience, and environmental and physiological conditions.
With regard to survey heights, IAGSA provides the following operational context in its Safety Policy Manual:
Pilots are often reminded of the increased risks associated with low flying. We can all recognize that flying a given mission at a lower altitude will aggravate the consequences of mechanical malfunctions or human error. Yet, it can be equally said that decreasing the survey height may improve the quality of certain kinds of geophysical data – and the acquisition of high quality data is, after all, the fundamental objective of geophysical survey flights. While thousands of safely flown survey hours confirm the industry’s ability to reconcile the needs of flight safety and data acquisition, minimum safe survey heights continue to be a cause for concern.
The idea of a fixed minimum safe survey height was vigorously debated, but evidently, no single universal “minimum safe survey height” can be designated given the wide variety of survey conditions and aircraft characteristics.Footnote 57
However, even with some debate concerning a minimum safe survey height, IAGSA recommends that:
Clients specify the maximum clearance height possible, consistent with the objectives of the survey to be flown and that operators, prior to commencing a survey, conduct a detailed risk analysis in accordance with an internationally recognized procedure considering, but not limited to, the following factors […]:
- terrain relief, elevation & vegetation canopy thickness
- aircraft type
- aircrew flight and duty times
- prevailing weather conditions
- anticipated density altitude
- pilot experience and recency
- planned flight speedFootnote 58
Subsection 2.3.2 of the Manual states:
Although helicopters can operate at a wide range of height and velocity combinations the selection of survey heights and speed can impact safety. Flying the helicopter at a lower height reduces safety margins from a CFIT perspective and the combination of lower survey speed and lower height may also place the helicopter in the avoid area of its published height-velocity diagram, making a successful autorotation unlikely in the event of an engine failure.Footnote 59
Section 2.4 of the Manual highlights the hazards of flights over water and cautions that “extra attention is required on height/altitude information because depth perception is often very poor, particularly in overcast and/or calm sea conditions.”Footnote 60 IAGSA also recommends that “single engine piston aircraft should not be used for over water/offshore surveys” and that aircraft should be equipped with “a radio or radar altimeter with a means of alerting the crew when height above the water falls below a minimum safety height selected by the crew.”Footnote 61
1.18.5.2 Flight Safety Foundation’s Basic Aviation Risk Standard program
The FSF has developed the Basic Aviation Risk Standard (BARS) as an international aviation safety program designed to provide a standard to assist in the risk-based management of aviation activities. The FSF designed the program to suit any organization that uses aircraft operators to provide contracted aviation support for its operations. It is a risk-based model framed against the actual threats posed to aviation operations that links threats to associated controls and recovery and mitigation measures. The BARS Implementation Guidelines manual and the Contracted Aircraft Operations manual both state:
Airborne geophysical survey operations are higher risk than other aviation activities in the resource sector. All proposed activities are subject to a detailed risk analysis that meets the standards of the company, aircraft operator and the IAGSA.Footnote 62,Footnote 63
These manuals provide minimum requirements for companies and operators to understand and mitigate the risk in their operations. An overview of the threats, associated controls, and recovery measures for airborne geophysical survey operations are provided in the BARS Bow Tie Risk ModelFootnote 64 (Appendix A) with relevant examplesFootnote 65 included (Appendix B).
Novatem and Synergy were not members of IAGSA or the FSF at the time of the occurrence, and it could not be determined if they had access to the aforementioned guidance. The investigation found no evidence of input from the expertise of industry agents, such as IAGSA or FSF, for the development of guidance documentation, procedures, training, or recommended aircraft modifications that could have supported Synergy’s transition to new operations.
1.18.6 Previous TSB investigation reports
1.18.6.1 Controlled flight into terrain due to glassy water
The TSB has investigated several occurrences in which flight over glassy water resulted in an accident. A search of the TSB’s Aviation Safety Information System (ASIS) database identified 13 helicopter occurrences (including this one) in Canada from 1988 to 2022 in which the loss of visual references due to flight over glassy water was a likely causal or contributing factor. Footnote 66 Of those, 5 occurrences resulted in 6 fatalities.
1.18.7 TSB Watchlist
The TSB Watchlist identifies the key safety issues that need to be addressed to make Canada’s transportation system even safer.
Safety management is a Watchlist 2022 issue. There are still no regulatory requirements for formal SMS for CARs Subpart 702 operators. As this occurrence demonstrates, SMSs that are implemented voluntarily by operators are not subject to TC regulatory oversight and may not be effective at identifying aviation hazards and managing their risks.
ACTION REQUIRED Safety management will remain on the Watchlist for the air transportation sector until:
|
2.0 Analysis
In this occurrence, the pilot held the appropriate licence and qualifications required for the flight in accordance with existing regulations, and there was no indication that his performance had been degraded by fatigue. In addition, there was no indication that the helicopter had experienced a system malfunction that could have contributed to the occurrence.
The analysis will examine the geophysical survey flight profile, the circumstances surrounding the occurrence flight, risk-mitigation factors, operating pressures, survival aspects, and issues with voluntary safety management systems (SMSs).
2.1 Geophysical survey flight profile
The geophysical survey task required intentional and precision low-level flying along predetermined survey lines over land and water to capture specific geomagnetic data. Given the new Novatem technology and equipment installed on the helicopter, the flight profile required that pilots consistently maintain the optimal height of approximately 25 m (82 feet) along each survey line; however, this was not clearly communicated to pilots. This implied that pilots fly the contour of the terrain. Speed was not a parameter that affected the quality of the data and was left to the pilot’s discretion.
Synergy Aviation Ltd. (Synergy) developed the Guimbal Aeromagnetic Survey Procedure (GASP) document to provide its pilots with the knowledge required to safely conduct the geophysical survey task. However, the GASP did not include any information on the flight profile parameters with respect to the optimal survey height or how to fly the contour of the terrain to keep the height consistent regardless of the surface being overflown. The investigation revealed that most Synergy pilots, including the occurrence pilot, were instructed verbally by the chief pilot to fly as low as safely possible, and within their own personal comfort limits, while flying the contour of the terrain.
The minimal information in the GASP, the verbal guidance that left pilots to select a safe height, and the absence of precise height indication on board resulted in pilots flying too high in some survey blocks, which resulted in poor-quality data. When this occurred, Novatem technicians asked pilots to fly lower. This led the pilots to rely almost exclusively on feedback from the technicians to visually adjust the height of their flight profiles without knowing the actual height of their flight because of the absence of a precise height indication on board.
Novatem informed the occurrence pilot 2 days before the occurrence that the survey data from 05 and 06 October showed that he was, on average, 10 m (32 feet) above the survey heights flown by the previous pilot and that the survey height should be lowered.
Flight data, recorded by Novatem's aeromagnetic survey equipment and Spidertracks, from flights other than the occurrence flight, were reviewed and indicated that pilots generally tended to descend to lower heights once they were flying over water. Novatem data indicated an average height of 12 m (approximately 40 feet) over water compared to an average height between 23 to 25 m (75 to 82 feet) over land. In comparison, Spidertracks data showed a similar pattern of flying between 6 to 12 m (approximately 20 to 40 feet) over water and 18 to 24 m (approximately 60 to 80 feet) over land.
The investigation determined that the Synergy pilots descended to these heights when flying over water instead of maintaining the same height over both water and land in response to client instructions and feedback regarding poor-quality data obtained when flying at the same height over land and water. The client instructions to fly as low as possible led the pilots to fly lower when over water or flat, open fields. As a result, the pilots would descend and, perhaps unknowingly, place themselves and the helicopter in a flight profile of increased risk.
The routine practice of descending to lower heights over water increases the risk of controlled flight into terrain (CFIT). If an unforeseen situation, such as a system malfunction or an inadvertent descent, arises at a low height, there may be insufficient height or time available for the pilot to recognize the situation and manage a recovery.
Finding as to causes and contributing factors
The routine practice of descending and flying below 25 m (82 feet) when over water or flat, open fields left the occurrence pilot insufficient height or time to detect and react to operational hazards.
2.2 Occurrence flight
2.2.1 Glassy water conditions
Environmental conditions over the lake at the time of the occurrence were reported as calm winds, no fog, and unlimited visibility. A photo of the lake taken about 1 hour after the accident shows patches of glassy water.
Glassy water conditions occur over water when the combination of wind and water movement produces no waves. This results in a mirror-like surface that creates a visual illusion and reduces visual cues for depth perception, which can impair a pilot’s ability to visually judge the aircraft’s height above the water. With diminished visual cues (surface features), depth perception can be reduced or lost completely. The reduction or loss of depth perception adversely affects a pilot’s spatial awareness and increases the risk of CFIT. The hazard of glassy water conditions is further exacerbated because the reduction or loss of depth perception often goes unnoticed, in part because visibility in this situation is good. In the absence of risk mitigation, the recognition of glassy water conditions in the flying environment is the only way pilots can take proper action to prevent CFIT caused by the insidious nature of the illusion and the loss of depth perception cues.
Training that incorporates operational hazards and effective mitigations is one way to improve awareness and detection of glassy water hazards. Synergy provided training to its pilots in preparation for the geophysical survey task over water; however, the glassy water hazard was not covered. The occurrence pilot was knowledgeable about the condition of glassy water but did not have experience managing the hazard in flight, specifically in geophysical survey operations (i.e., low-level flying).
2.2.2 Undetected descent
During the occurrence flight, the pilot flew mostly over undulating, forested terrain, which provided the pilot with adequate visual cues required for depth perception and, thus, the ability to judge and maintain height. When flying over water, the pilot used the surface of the lake, the shoreline, and the trees as visual references. On the day of the occurrence, glassy water conditions were present, and the pilot’s ability to judge and maintain height was reduced. Due to the glassy water hazard, the insidious reduction of visual cues needed for depth perception went unnoticed by the pilot.
The last 2 recorded heights by the Spidertracks system were 26 feet (7.9 m) at 1139 and 42 feet (12.8 m) at 1141 while the helicopter was over water. After the 180° turn for the northbound leg, the aircraft was evaluated to be approximately 1200 feet from the western shoreline. While this distance may not seem far, the low operating height likely made it difficult for the pilot to use the shoreline and trees to assess, determine, and maintain the helicopter’s height over the water. During that last manoeuvre, the pilot corrected the helicopter’s lateral spacing and while doing so, an inadvertent descent went unnoticed by him.
Given the low level at which the pilot was operating, he had little time or height available to detect and respond to hazards and undesired aircraft states. With an insufficient number of visual cues for the pilot to accurately judge his height over water, and with no equipment providing precise height indications or alerts to the low heights, the helicopter inadvertently descended during or after the 180° turn and course correction over the glassy water.
Finding as to causes and contributing factors
While flying at low level over the lake, the mirror effect of the glassy water resulted in a reduction of visual cues needed for depth perception. Consequently, an inadvertent descent went unnoticed by the pilot and the helicopter collided with the surface of the lake.
2.3 Risk mitigation
It is unrealistic for operators to identify all potential hazards and risks that could be encountered during operational flights at the beginning of a new contract or operation. SMSs support the development of operational knowledge and continuous improvement of training and procedures.
Synergy adopted several safety measures to mitigate the operational pressures, including:
- Policies and procedures: created the GASP and implemented a flight-following system.
- Helicopter modifications: installed emergency flotation equipment for over-water operations.
- Training: provided training on underwater egress and flying survey profiles.
- Safety equipment: provided personal flotation devices (PFDs).
However, gaps in procedures, training, equipment, and regulatory guidance existed at the time of the accident.
2.3.1 Procedures and training
It is important that information be communicated in guidance documentation, including the company operations manual (COM), that knowledge taught during training be clear, complete, and accurate, and that training be as practical and realistic as possible. To assist operators with determining safe operating practices, several sources of information are available, such as publications provided by the International Airborne Geophysics Safety Association and the Flight Safety Foundation. The investigation did not reveal any evidence of input from other industry agents adding knowledge to the new operation.
The procedures developed for this contract were included in the GASP. However, the COM was not amended to include these procedures related to the new aerial work associated with the geophysical surveys. The GASP listed some hazards related to low flying but did not provide guidance on how to mitigate them. Information about the hazard of glassy water was not documented in the GASP or the COM.
A post-occurrence amendment to the GASP directed that “[a]ll flights shall be conducted no lower than 200ft AGL [above ground level]” [emphasis in original]. However, the optimal height required by the aeromagnetic survey equipment for valid data remained at 25 m (82 feet), even with this new directive. The updated GASP did not resolve the discrepancy between the training height of 200 ft AGL and the optimal height of 25 m (82 feet).
The in-flight training was conducted without the aeromagnetic survey equipment installed because an instructor was required on board and sat in the left seat, where the equipment had to be installed. This meant that pilots only used the aeromagnetic survey equipment once they were deployed on site, during actual operational flights. Also, the in-flight training was conducted at heights and in a geographical location that were not representative of the flight profile or the environment in which the survey flights would take place.
The combination of these factors left the pilot with insufficient knowledge and guidance to mitigate the hazards and risks of the aeromagnetic survey operations in the low-level and over-water environment.
Finding as to risk
If company procedures for operations in low-level and over-water environments do not contain information related to the specific hazards and mitigation strategies related to these operations, there is a risk that company pilots will not have a comprehensive understanding of the risks or take steps to mitigate them.
2.3.2 On-board equipment and technology for low-level operations
Low flying reduces the height or time available to recognize and manage unexpected events, such as inadvertent descents. From 1988 to 2022, the TSB has investigated 13 helicopter occurrences in which a loss of visual references due to flight over glassy water was a likely causal or contributing factor.
The use of technology to provide height and altitude information to pilots, including alerts when the height falls below a minimum threshold, helps manage the risk of CFIT. In this occurrence, the only instrument that provided altitude information was the barometric altimeter, which did not provide precision height indications or any visual or aural alerts to the pilot. This left the pilot to rely only on his visual cues to determine and maintain his height during the low-level flights.
Finding as to risk
If helicopters are operated at low level in areas where there are insufficient visual cues to accurately determine height above ground, and additional equipment is not installed or used to help determine the helicopter’s height, there is an increased risk that an inadvertent descent will result in a collision with terrain.
2.3.3 Guidance for developing a company operations manual
The Canadian Aviation Regulations (CARs) requirements relating to COMs for aerial-work operations state that a COM shall be submitted to Transport Canada (TC) and, if there are any changes to the company’s operations, the air operator shall amend its COM. While the development of the GASP was an attempt to meet the CARs and Commercial Air Service Standards (CASS) requirements, it was not included as an amendment in the COM and, as a result, TC was unaware of its development or content and therefore was unable to provide any oversight.
A review of the CASS requirements shows that, while there are specific operational details that must be included in a COM for external load operations, requirements for other aerial-work operations, such as low-level operations or flight over water, are less strict. Standards for those operations only stipulate “low level flight precautions” Footnote 67 and “operational restrictions related to aerial work operations.” Footnote 68
Given the inherent hazards and risks present when operating in low-level or over-water environments, additional information on altitude restrictions, safe operating altitudes, operational procedures, and equipment specifications for the conduct of low-level flight or flights over water can support safer operations and the management of risks of CFIT by air operators and their pilots. The minimal requirements in the CASS may be contributing to insufficient details in procedures for aerial-work operations conducted in low-level and over-water environments.
Finding as to risk
If the CASS do not require a COM to include specific operational information and hazards related to flight in low-level and over-water environments, there is a risk these will not be considered when companies develop operating procedures and mitigation strategies.
2.4 Operational pressures and acceptance of unsafe practices
CARs Subpart 702 (Aerial Work) operators face operational pressures that must be identified and managed so that they can deliver a service and stay safe, while also remaining economically viable. The goal is to keep the air operation safe by managing these operational pressures; however, “getting the job done” is also seen as a primary goal. When operational pressures become unbalanced, an operation can be pushed beyond safety limits. When operational pressures are not identified or are mismanaged, an organization or its people may accept unsafe practices, increasing the risk of an incident or accident.
The actual task of geophysical survey work is inherently risky because it requires pilots to fly very precise flight profiles at low heights for extended periods of time. This requires considerable skill and knowledge of the hazards and risks present during low-level operations. In addition, the specific equipment used by Novatem required that the occurrence helicopter be flown and maintained at a height considerably lower than that required by other airborne geophysical survey systems.
In this operation, Synergy and its pilots were faced with the following pressures:
- Novatem’s requirement to obtain quality data;
- Synergy’s direction to fly low;
- Relative low number of flight hours/inexperience of the pilots; and
- Pilots’ individual pressure.
To deal with this new operation, Synergy implemented several safety measures related to policies and procedures, helicopter modifications, training, and safety equipment.
Even though these safety measures were there to address the operational pressures, they did not provide pilots with the information they needed to conduct the task safely. Specifically, the GASP did not contain information on optimal heights to be flown, contour flying, and consistency in maintaining the optimal height (25 m [82 feet]) on all survey lines—the most important technique for obtaining good-quality data. With limited experience in flying this type of operation, client pressure to obtain quality data, and the operator’s direction to fly as low as safely possible within their own personal limits, company pilots were found in the precarious position to fly at heights that were below those at which they had been trained.
The investigation determined that while several pilots were often uncomfortable with the required low flight profile and shared their concerns with the chief pilot, they continued to fly at the required low heights to accomplish the task. Given the pilots’ individual pressure to satisfy both the client and their employer, the operation saw a shift to the acceptance of unsafe practices such as not flying a consistent contour profile over terrain, which resulted in flying below the operational height required for the survey flights. As the contract work continued, and with the positive outcomes of successful flights and obtaining quality data, flying lower than necessary became the accepted practice.
Finding as to risk
If air operators do not implement safety measures that manage operational pressures, there is a risk that pilots will develop, and company management will accept, unsafe practices that can lead to incidents or accidents.
2.5 Survival aspects
2.5.1 Flight following
Synergy was not required to implement flight following for its day visual flight rules (VFR) single-pilot operations. However, it had a flight-following system in which junior pilots were tasked with monitoring all the operational flights with the Spidertracks flight-tracking system.
On the day of the occurrence, the flight follower did not detect that the helicopter was stationary on the display from the time of the accident at 1141 until Novatem called Synergy at 1246 to request an update on the helicopter’s flight status. In addition, no tier one alert was raised from the Spidertracks emergency management framework because the organizational settings were set to normal mode instead of watch mode. In watch mode, an alert is automatically activated if communication with the aircraft is lost for a period of 10 minutes.
Finding as to risk
If company flight-following systems are used without optimizing all available operating modes, there is a risk that the activation of search and rescue services will be delayed in the event of an accident.
2.5.2 Personal flotation devices
The pilot wore a TC Marine–approved PFD that inflated automatically when it was submerged after the impact.
A review of the standards and requirements of the CARs indicates that a PFD that is intended for use in an aviation environment must meet the manual-only inflation criterion. The PFD worn in this occurrence was fitted with a hydrostatic inflator; therefore, it did not meet this requirement.
In this occurrence, once the PFD had inflated inside the helicopter, the pilot experienced difficulty with his egress due to restrictions in mobility and increased buoyancy. The inflated PFD further complicated the detaching and untangling of the shoulder harness straps and helmet communications cord. These hazards can hamper or prevent a successful egress, and increase the risk of drowning.
Finding as to risk
If aircraft occupants wear PFDs that do not meet the CARs standards and requirements for use in aviation, there is a risk that, in the event of a crash into water, the PFD will hamper or prevent a safe underwater egress.
The PFD assisted the pilot in reaching the surface of the water, at which point it supported his weight and provided adequate flotation, allowing him to swim to the nearest shore in the cold water.
Finding: Other
Wearing a PFD increased the pilot’s chances of survival by helping him stay afloat during his 40-minute swim to shore.
2.5.3 Helmets, lap straps, and shoulder harnesses
The pilot was wearing his helmet, lap strap, and shoulder harness.
The pilot reported a sudden, rapid longitudinal deceleration followed by a forceful water ingress. Although the pilot sustained serious injuries during the impact sequence, his helmet provided head protection and the lap strap and shoulder harness properly restrained him. The use of this safety equipment likely contributed to his ability to conduct a successful egress.
Finding: Other
Wearing a flight helmet and fastening both the lap strap and the shoulder harness helped to reduce the severity of the injuries to the occurrence pilot during the impact sequence.
2.5.4 Emergency flotation equipment
The occurrence helicopter was fitted with emergency flotation equipment that is designed to inflate automatically (without any pilot input) when an immersion is detected, if the system is armed with the arming switch.
In this occurrence, the floats did not deploy after the impact with the water. Due to the unexpected nature of the impact, the pilot reported not having time to manually inflate the system. The investigation was unable to determine the position of the arming switch or whether the system malfunctioned due to the impact sequence.
Finding: Other
For undetermined reasons, after the helicopter’s impact with the water, the emergency flotation equipment did not deploy. Keeping the helicopter afloat would have allowed the pilot to egress on the water’s surface, and facilitated the search for the aircraft.
2.5.5 Underwater egress training
Underwater egress training provides pilots with the skills, knowledge, and confidence needed to survive real-life escape situations, such as a crash or ditching into water. In this occurrence, the pilot was facing a real-life escape situation as the helicopter was inverted and submerged in cold water. This situation required an immediate, rapid response from the pilot to egress safely and avoid being trapped inside the helicopter and drowning.
Finding: Other
Underwater egress training provided the pilot with the skills required to safely egress a submerged and inverted helicopter.
2.6 Transport Canada safety management systems
Many CARs Subpart 702 operators are taking a proactive approach to safety by identifying and mitigating risks associated with their activities, and a number of these operators are taking measures that exceed regulatory requirements by implementing an SMS. Synergy implemented an SMS even though it was not required by regulation.
Before the occurrence, several pilots had verbally raised concerns with the chief pilot about the low heights required for the survey flights. They deemed the flight profile risky and were often uncomfortable with these low heights. However, the investigation did not reveal any entries or follow-ups in the online SMS reporting tool from pilots concerning low-level flight or unsafe conditions in the geophysical survey operation.
TC does not monitor or regulate SMSs for operators that are not required to have one. As a result, the effectiveness of these SMSs for managing aviation safety cannot be determined. Although the TSB recommended to TC in 2016 that all commercial aviation operators in Canada implement a formal SMS, it was not until March 2023 that the Board considered TC’s response to Recommendation A16-12 to be Satisfactory in Part. Before that, the Board was unable to assess TC’s responses to the recommendation because TC was not clear on how the initiatives taken would address the safety deficiency identified in the recommendation.
The issue of safety management has been on the TSB Watchlist since 2010. As highlighted in Watchlist 2022, progress has been slow to extend the application of SMS beyond CARs Subpart 705 operators. While the Board is encouraged that TC is conducting an SMS policy review that will assist in developing recommendations to modernize and expand SMS requirements, until this review is complete and changes are implemented to the SMS requirements, it is unclear whether these efforts will address the safety deficiency identified in the recommendation.
Finding as to risk
If TC does not mandate and oversee SMSs for all commercial operators, there is a risk that the systems that are voluntarily implemented will not be effective at managing aviation hazards and risks.
3.0 Findings
3.1 Findings as to causes and contributing factors
These are conditions, acts or safety deficiencies that were found to have caused or contributed to this occurrence.
- The routine practice of descending and flying below 25 m (82 feet) when over water or flat, open fields, left the occurrence pilot insufficient height or time to detect and react to operational hazards.
- While flying at low level over the lake, the mirror effect of the glassy water resulted in a reduction of visual cues needed for depth perception. Consequently, an inadvertent descent went unnoticed by the pilot and the helicopter collided with the surface of the lake.
3.2 Findings as to risk
These are conditions, unsafe acts or safety deficiencies that were found not to be a factor in this occurrence but could have adverse consequences in future occurrences.
- If company procedures for operations in low-level and over-water environments do not contain information related to the specific hazards and mitigation strategies related to these operations, there is a risk that company pilots will not have a comprehensive understanding of the risks or take steps to mitigate them.
- If helicopters are operated at low level in areas where there are insufficient visual cues to accurately determine height above ground, and additional equipment is not installed or used to help determine the helicopter’s height, there is an increased risk that an inadvertent descent will result in a collision with terrain.
- If the Commercial Air Service Standards do not require a company operations manual to include specific operational information and hazards related to flight in low-level and over-water environments, there is a risk these will not be considered when companies develop operating procedures and mitigation strategies.
- If air operators do not implement safety measures that manage operational pressures, there is a risk that pilots will develop, and company management will accept, unsafe practices that can lead to incidents or accidents.
- If company flight-following systems are used without optimizing all available operating modes, there is a risk that the activation of search and rescue services will be delayed in the event of an accident .
- If aircraft occupants wear personal flotation devices that do not meet the Canadian Aviation Regulations standards and requirements for use in aviation, there is a risk that, in the event of a crash into water, the personal flotation device will hamper or prevent a safe underwater egress.
- If Transport Canada does not mandate and oversee safety management systems for all commercial operators, there is a risk that the systems that are voluntarily implemented will not be effective at managing aviation hazards and risks.
3.3 Other findings
These items could enhance safety, resolve an issue of controversy, or provide a data point for future safety studies.
- Wearing a personal flotation device increased the pilot’s chances of survival by helping him stay afloat during his 40-minute swim to shore.
- Wearing a flight helmet and fastening both the lap strap and the shoulder harness helped to reduce the severity of the injuries to the occurrence pilot during the impact sequence.
- For undetermined reasons, after the helicopter’s impact with the water, the emergency flotation equipment did not deploy. Keeping the helicopter afloat would have allowed the pilot to egress on the water’s surface, and facilitated the search for the aircraft.
- Underwater egress training provided the pilot with the skills required to safely egress a submerged and inverted helicopter.
4.0 Safety action
4.1 Safety action taken
4.1.1 Synergy Aviation Ltd.
After the occurrence, a safety management system (SMS) investigation and report were completed, a series of corrective actions were identified, and the company continues to monitor their implementation.
A post-occurrence amendment to the Guimbal Aeromagnetic Survey Procedure (GASP) was incorporated and included a single statement: “All flights shall be conducted no lower than 200ft AGL [above ground level].” [emphasis in original]
This report concludes the Transportation Safety Board of Canada’s investigation into this occurrence. The Board authorized the release of this report on . It was officially released on .
Appendices
Appendix A – Basic Aviation Risk Standard Bow Tie Risk Model
Source: Flight Safety Foundation, Basic Aviation Risk Standard - Contracted Aircraft Operations, Version 8 (May 2020), p. 44.
Appendix B – Basic Aviation Risk Standard Airborne Geophysical Survey Operations
The following table lists some threats, controls, safety goals, and defences identified in the Basic Aviation Risk Standard (BARS) Airborne Geophysical Survey Operations program.
Threat | Control | Safety goal | Defence |
---|---|---|---|
Personnel | Aircrew experience and recency | Ensuring flight crew are competent to conduct survey operations duties by having appropriate training, qualifications, and recent experience. | Required experience, flight hours, and training for pilots. |
Personnel | Flight crew remuneration | Ensuring that the safety of survey operations is not compromised by unnecessary pressure on flight crew. | To remove unnecessary pressure to fly and potentially compromise minimum standards, flight crews must not be paid on the basis of hours or distance flown. |
Personnel | Crew complement | Ensuring crew composition planning is cognizant of the high workload of survey flying and is appropriately managed. | The minimum crew complement must be a pilot and operator. Single-pilot operations must only be accepted after conducting a risk assessment that delivers mitigation measures acceptable to all. Where an observer is carried due to operating country requirements, the observer is to be considered part of the crew. |
Aircraft equipment | Radar altimeter | Ensuring the provision of reliable radar altimeter data and warnings to provide clear and reliable awareness of height above terrain/water. | Equip the aircraft with either a dual output radar altimeter or 2 independent radar altimeters, fitted with visual and aural height warnings, and with a variable height alert that can be set by the crew. |
Survey flight operations | Risk assessment | Ensuring all risks associated with geophysical operations are analyzed, minimized, and accepted. | Aircraft operator performs and documents a risk assessment that has been reviewed by a competent aviation specialist and has involved all relevant stakeholders including flight crew. |
Survey flight operations | Minimum survey heights | Ensuring surveys are conducted at a safe height after consideration of all factors including terrain and aircraft type. | The survey height is defined as the height above obstacle level, such as the top of a jungle canopy in a tropical environment or ground level in desert conditions. Where the survey height is nominated below 100 m for fixed wing, 60 m for helicopters or 50 m for a towed object, approval must be based on a risk assessment and agreed by all parties. |
Survey flight operations | Survey speed – rotary-wing aircraft | Ensuring appropriate survey speeds are calculated for rotary-wing aircraft to allow safe control margins. | With the exception of takeoff and landings, helicopters must minimize flight inside the avoid curve of the published height-velocity diagram or below single-engine fly-away speed for multi-engine helicopters. Where operations in this flight regime are unavoidable due to the type of survey and equipment, conduct a risk assessment including an assessment of the terrain. |
Survey flight operations | Turning radius | Ensuring appropriate limitations on aircraft turns during surveys. | Limit turns at low level to a maximum angle of bank of 30° and conduct them at a constant altitude. If the aircraft must climb due to the surrounding terrain, it should climb to the required height before commencing the turn. Descent back to survey height must only occur after wings-level attitude is established. |
Survey flight operations | Performance monitoring | Ensuring compliance with minimum survey parameters. | Performance parameters including aircraft speed, height above terrain and drape must be periodically reviewed using data collected during the survey. Inspect deviations below minimum survey speed and minimum height. Take corrective actions to ensure deviations cease and the minimum safety margins are maintained. Determine the frequency of performance parameter reviews during the pre-start risk assessment. |
Compiled from text and information presented in: Flight Safety Foundation, Basic Aviation Risk Standard - Contracted Aircraft Operations, Version 8 (May 2020), pp. 45-50.